Merry Christmas and Happy Hannukah to all! I apologize if this is weird timing, but I’ve been working on this post for like 2 weeks, and I don’t like waiting to get posts out. Hope you enjoy regardless.
Reading that the UnitedHealth Group assassin was possibly radicalized by his back pain has got me thinking about pain again. Pain is bad, basically by definition. It’s shocking how bad it can be. We forget how bad it is once we’re no longer in pain, but, in the moment, getting stung by a hornet, stubbing a toe, or suffering the aftereffects of mall sushi is mind-numbing. It’s impossible to think about anything else.
I’ve generally been pretty lucky with regards to avoiding pain, except for some unfortunate digestive issues in my early to mid 20s (a guide to treating them was actually one of my first viral posts). Still, the topic fascinates me. Pain is likely the oldest medical problem. We’ve been seeking out remedies for it for millenia, from alcohol to willow bark. Even orangutans try to self-medicate for it.
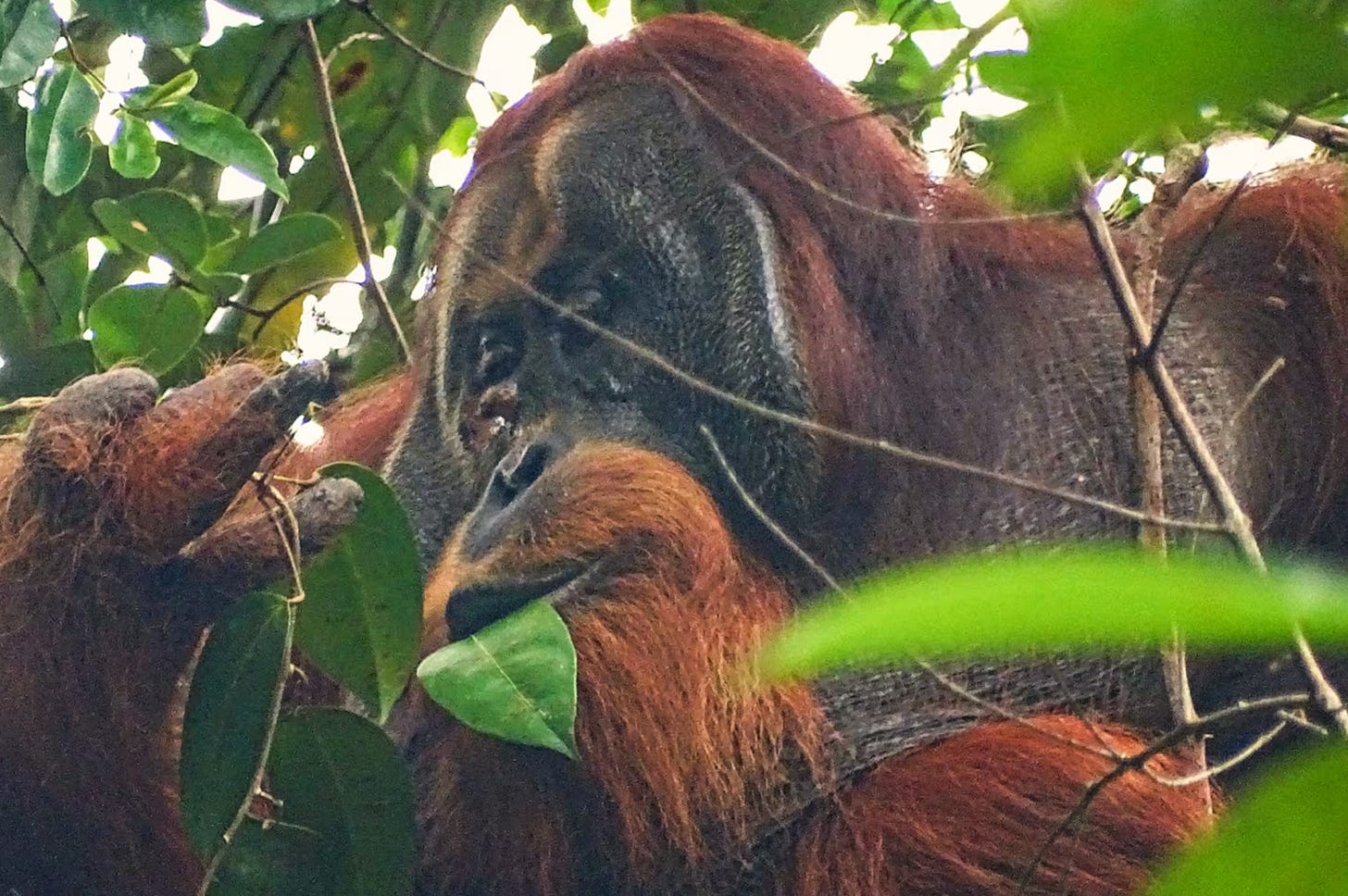
And yet, still, we don’t really understand how pain functions, what causes it, or how to stop it in a precise manner. Our best tools for diagnosing pain have not really progressed beyond asking people if they have pain, or, in the case of animals, observing them and seeing if they act like they’re in pain. Our best tools for stopping pain are either diffuse global treatments with tons of side effects, like opioids and NSAIDs, or blunt force instruments directly to the nervous system, like nerve blocks. Despite our best efforts, we still lack any way to tell objectively if a person or animal is in pain, and any way to stop pain while leaving the rest of the body unaffected.
A big part of this is because of how fundamental pain is to bodily functioning. Every organism feels pain in some way or another because it’s a basic signal that something is causing harm to them. Even paramecium will flee noxious stimuli in a not so different way than a person quickly withdrawing their hand from a hot stove.
For humans and other higher-order animals, pain is integrated into every part of our body, making it hard to block or measure. First, many types of stimuli can cause pain, from the mechanical, like hitting your thumb with a hammer, to the chemical, like a bee sting, to the purely physiological, like “pins and needles”. Those stimuli interact with pain-signal-carrying nerve fibers differently, requiring nerve fibers to have a variety of ways of receiving pain signals.
Speaking of nerve fibers, multiple types of nerve fibers can carry pain signals, making for redundant, robust signals that can’t easily be blocked, which is probably for the best, considering the importance of the signal to tell us that something is wrong. For those parts of the body that are not innervated by pain-conducting nerve fibers, like the heart, primary sensory neurons can “refer” pain to the parts that can, like the infamous heart attack symptom of shooting arm pain.
Then, once the pain signals hit the brain, multiple parts of the brain are involved simultaneously with processing pain. This is why traumatic brain injuries can result in loss of smell, if the injury impacts the olfactory bulb, or sight, if it impacts the visual cortex, but almost never loss of pain. Even patients in a vegetative state can often still feel pain on some level.
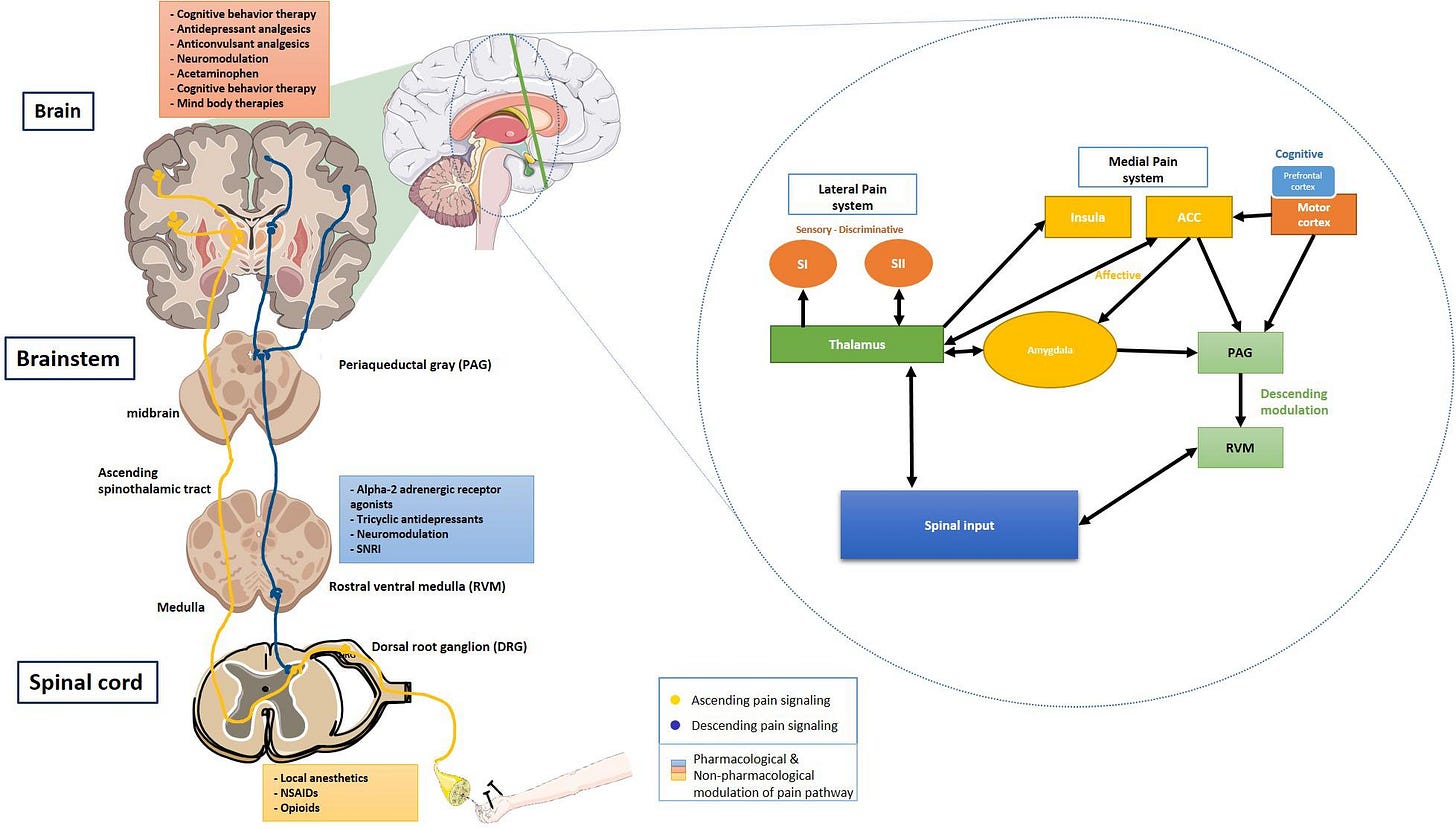
Pain itself can also be a purely mental phenomenon. Everyone has had the experience of stress causing cramps or headaches. And many people have reported successfully relieving themselves of back pain by following Sarno’s method of treating back pain as a purely mental phenomenon caused by stress, rather than as physical phenomenon caused by mechanical stressors. Pain is felt in the body, but it happens in the brain.
In the past, when I’ve tried to think about pain in a scientific way, the above is where I got stuck. It’s hard enough tracking pain through the nerve fibers, but once it gets into the brain, I get totally lost. I fully support anyone trying to work in the pain space, because it’s important, but it’s hard, and made harder by the involvement of the cops at the DEA.
I’ve decided to take a different tact in my thinking this time in hopes that I get less stuck. Instead of talking about pain in general, or even the sorts of pain I’ve experienced, I’m going to go back to a disease that I’ve talked about many times before: diabetes. One of the reasons diabetes remains so fascinating to me is that it can affect every possible part of the body in many different ways, including through pain.
Specifically, diabetes frequently causes peripheral neuropathic pain, which is not a particularly well-defined set of symptoms. Basically, it’s any pain that occurs on the feet or hands that doesn’t have an obvious mechanical cause, like a wound or mechanical compression. What makes this interesting is that this is almost a “pure” form of pain: it results directly from damage to the nerves, through reasonably clear mechanisms, and is barely affected by weak painkillers.
Diabetes, as regular readers of my blog know, has unclear beginnings. Type 1 diabetes is in full swing by the time pancreatic beta cells are being destroyed, and Type 2 diabetes is in full swing by the time cells start resisting the insulin signal, but what happens before that is shrouded in mystery. Fortunately, for diabetic pain, we can ignore that, as pain only happens when either type of diabetes is in full swing.
Once type 1 or type 2 diabetes is in full swing, the most important event happening in the body is high blood sugar. This is bad for nerves, specifically, because nerves have high energy requirements. They constantly have to maintain a polarized membrane, be ready to fire by depolarizing and repolarizing to propagate signals, and release and reuptake neurotransmitters1. This requires carefully orchestrated energy dynamics, in which glucose is taken up by the neuronal glucose transporter, GLUT1, shuttled into the neurons and astrocytes (neuronal helper cells), and carefully divvied up into either lactate, if shuttled into the astrocyte, or kept as plain glucose in preparation for the neuronal energy needs.
Hyperglycemia is bad because GLUT1 doesn’t expect there to be too much blood sugar. Normally, there’s barely enough glucose for all the neuronal needs, so GLUT1 will let as much in as exists outside the neuron. In the case of hyperglycemia, this means that GLUT1 lets in so much glucose that it all piles up in the extraneuronal space.
If left unchecked, this piling up of glucose triggers the mitochondria in the neurons to first hyperpolarize, as their electron transport chains run on overdrive, and then depolarize, as the reactive oxygen waste products from the transport chains build up. At that point, the mitochondria can no longer function, the neurons can’t get energy, and they start to die. Simultaneously, the excess glucose forms bonds with proteins and lipids through glycation, permanently altering the proteins and lipids and disrupting pretty much all processes that rely on them, including the electron transport chain.
This is bad. To avoid this, there are several last-ditch pathways to prevent this build up, which involve converting glucose into other, less harmful products, like sorbitol and UDP-GlcNAc2. These products, although less harmful than glucose, are still somewhat harmful, so they also cause damage as they build up.
Because the damage of hyperglycemia is predominantly an issue of interference with energy demands, the nerve cells that are affected first are those that have high energy needs: unmyelinated and thinly myelinated nerve fibers at the ends of long axons, like the feet and hands. This is universal among diabetes patients, which is why sensation is always lost in the extremities first.
Pain, by contrast, is not universal in diabetic patients. Nerve damage or even nerve death, in and of themselves, don’t cause pain. It’s only once the ion channels are modified in the nerves that pain can be felt. The “channel lost” neuropathic pain in diabetes comes from semi-permanent modification of the ion channels by SUMOylation3. This can only happen if the ion channels are relatively intact, which means the neurons haven’t gotten permanently depolarized yet.
So, at last, we can come to a reasonably robust way of how diabetics end up in pain. As hyperglycemia builds up, the excess glucose simultaneously causes the mitochondria to run on overdrive and jams up the proteins and lipids in the mitochondria. The nerve cells react by semi-permanently modifying their ion channels, which causes a pain signal to be sent every time the nerves fire by depolarizing and repolarizing, assuming the ion channels are still in good enough shape to fire. This can only happen for a limited amount of time, though, as eventually the mitochondria and the nerve fibers are in such bad shape that no firing can happen or ion channels can’t be modified any more.
If we wanted to directly target chronic pain in humans, finding ways to prevent semi-permanent modification of ion channels in nerve cells would be a good place to start. Acute pain relies on much quicker and more reversible modification of ion channels. The tricky part is that SUMOylation occurs everywhere, not just in nerve cells, and compounds that block it universally, like the acid from gingko biloba or cashew fruit, are either toxic or ineffective.
So, perhaps what we need is some way of localizing our blocking compounds to just neurons. Perhaps this would be a good place to start using adeno-associated viruses (AAVs) to carry an inhibition payload.
Past this point are my speculations on how to use AAVs as a precision method to stop a pain signal. The science of AAVs is way out of my comfort zone, so you’re not missing much if you stop reading here.
AAVs are small viruses that infect humans and primates. They are growing increasingly popular as ways to carry therapies, especially gene therapies, because they seem to be able to infect pretty much any cell, don’t cause major health consequences or immune responses, and can stably integrate into the host genome. A ton of work has been done on optimizing them for therapeutic purposes, which is way beyond the scope of this post and my knowledge base.
The important thing for us is that they can carry genetic payloads, such as RNA inhibitors, which, coincidentally, was the topic of another one of my early viral posts (no pun intended). We can optimize our AAV vector by choosing an AAV that specializes in invading neural tissues (Claude suggests AAV9), and modifying it to make sure it can only release its payload (i.e. express the genes it’s carrying) in neurons.
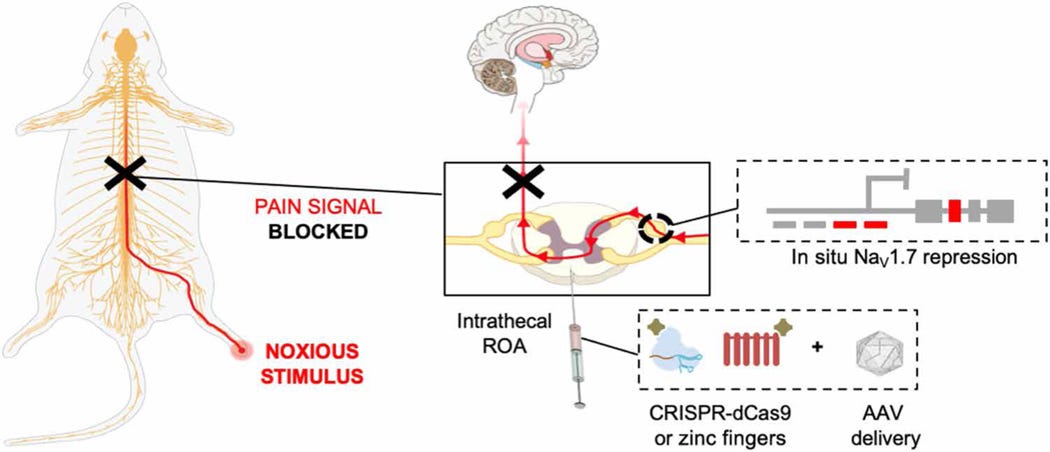
A single injection directly into the intrathecal space by the spine would likely be all we’d need for delivery. While this is a bit scarier than an intravenous injection, it is much more targeted, which means we wouldn’t risk introducing our virus into parts of the body it’s not supposed to go. Our best choice for an RNA inhibitor, meanwhile, would probably be a short hairpin RNA (shRNA), which we could get our nerve cells to continually produce.
We’d use our shRNA to mark our ion channel mRNA for destruction, so the ion channels would not be maintained by the cell and would disappear. This would be a bit dangerous, safety-wise: not only would we be getting rid of some or all of the pain response in our nerves (depending on the levels of shRNA), we’d also be continually devoting some large percentage of the nerve cell’s RNAi machinery to this task, possibly disrupting other important tasks that it needs to carry out. Careful targeting of exactly what we want to destroy, monitoring how much of it we’re destroying, and preserving the health of the overall nerve cell would be crucial for success of this.
But, if we succeed, we’d have a treatment for diabetic neuropathic pain that’d be more effective than anti-inflammatories, safer than opioids, and more sustainable than a nerve block. And that, in turn, might serve as a better model for how to treat back pain than surgery or, you know, patients taking matters into their own hands.
A lot of my perspective on diabetic neuropathy has been shaped by this review paper. Most of my sourcing for this section comes from here.
This may be the worst acronym I’ve ever seen. The full name is even harder to pronounce: Uridine diphosphate N-acetylglucosamine.
Way less fun than it sounds. SUMO are small ubiquitin-like modifiers.
Kratom is reportedly better than opiates: less addictive, less side effects….